- CSCD核心中文核心科技核心
- RCCSE(A+)公路运输高质量期刊T1
- Ei CompendexScopusWJCI
- EBSCOPж(AJ)JST
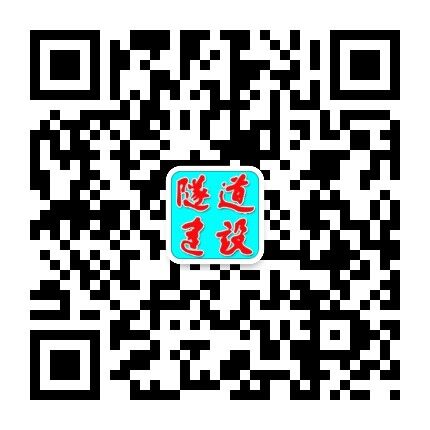
隧道建设(中英文) ›› 2024, Vol. 44 ›› Issue (7): 1440-1453.DOI: 10.3973/j.issn.2096-4498.2024.07.011
李飞龙1, 2, 3, 姜昌山2, 3, 余虔2, 3, 韩进宝2, 3, 张合青2, 3, 骆建军1, *, 王锦华2, 3
(1. 北京交通大学土木建筑工程学院, 北京 100044; 2. 民航机场规划设计研究总院有限公司, 北京 100029; 3. 机场工程安全与长期性能交通运输行业野外科学观测研究基地, 北京 101312)
Aerodynamic Effect Characteristics of Various Tunnel Lining Structures Passed by High-Speed Trains
LI Feilong1, 2, 3, JIANG Changshan2, 3, YU Qian2, 3, HAN Jinbao2, 3,
ZHANG Heqing2, 3, LUO Jianjun1,
*, WANG Jinhua2, 3
(1. School of Civil Engineering, Beijing Jiaotong University, Beijing 100044, China; 2. China Airport Planning & Design Institute Co., Ltd., Beijing 100029, China; 3. Observation and Research Base of Transport Industry of Airport Engineering Safety and Long-term Performance, Beijing 101312, China)
摘要: 为研究隧道衬砌结构上的气动压力时空特征和不同影响因素下气动效应的变化规律,基于滑移网格技术、有限体积法理论以及非定常、黏性、可压缩性N-S方程和RNG 湍流方程,建立精细化的隧道-列车流固耦合数值计算模型,通过动模型试验验证数值计算的合理性。研究表明: 1)全过程压力云图和流场云图可清晰地揭示出气动压力波的传播过程及特性; 2)基于列车长度修正了最大压缩波的既有经验公式,提出列车摩阻因数为2.255 Pa/m; 3)拟合出了不同隧道衬砌结构下压力峰峰值衰减率与循环周期数的关系式,为后期分析气动荷载对衬砌结构的疲劳损伤奠定了理论基础; 4)揭示出列车尾部完全驶出隧道后或长隧道中管片结构上的气动压力峰值相较于模筑衬砌隧道中的气动压力峰值衰减更快,盾构隧道出口外20 m和50 m处的微气压波幅值相较于模筑衬砌隧道降低1.53%~5.5%。